It seems straightforward: Cool natural gas to a low enough temperature and there you have it – the coveted LNG, which is set to play an even greater role in the energy mix in future. But it's not quite that simple. As is often the case, preparation is half the battle. And what's more, nothing can happen without explosion protection.
It's true that LNG (liquefied natural gas) is produced by liquefying natural gas by cooling (cryogenic treatment), but it has to be prepared first. Only the methane CH4, which is almost pure, is liquefied. By the end, this simplest of the hydrocarbons comprises 98 per cent of LNG. The methane content is often even higher than conventional natural gas in gas form. Such pure LNG is neither toxic nor corrosive.
Preparing raw natural gas
Prior to the actual liquefaction process, the raw natural gas needs to be cleaned, i.e. undesirable components need to be removed. Raw natural gas may contain numerous by-products, in particular
- lower hydrocarbons – ethane C2H5, (up to 15%), propane C3H8 (up to 10%), butane C4H10
- heavy hydrocarbons, BTEX (benzene, toluene, ethylbenzene and xylene)
- nitrogen N2
- hydrogen sulphide
- carbon dioxide CO2
and other components that are present in raw natural gas in different quantities depending on its origin. In particular, there are certain organic sulphur compounds which are undesirable in the end product and must be removed. Mercury is also present in raw natural gas in certain incidences, often in not insignificant quantities of several milligrams per cubic metre. Another undesirable component is water. It would be frozen during extreme cooling in the process sequence. What is known as "lean" natural gas with a very low methane content of around 30% can only be liquefied into LNG if other fractions are removed beforehand.
In principle, all components that have a poisonous or corrosive effect must be separated from the raw natural gas. Non-combustible components are also mostly removed. This includes noble gases, as well as water. Separating the by-products automatically increases the methane content. Some of the separated components, such as certain hydrocarbons, sulphur or mercury, can be used elsewhere. This also includes the valuable noble gas helium, which forms up to 7% of some natural gases.
Separating contaminants and by-products
Adsorption, absorption, gas scrubbing with particular solvents and cryogenic rectification, among other processes, are suitable for separating out undesirable trace substances. In the following, we will explain the most important steps for removing contaminants.
Step 1: Removing water and heavy hydrocarbons (gas condensates)
Various processes are used to dehydrate natural gas, during which heavy hydrocarbons are also sometimes separated:
- Absorption in triethylene glycol (TEG): Natural gas is conducted through an absorption column. This highly hygroscopic TEG flows through as a countercurrent, a substance to which water and higher hydrocarbons bind.
- Dehydration using a molecular sieve: Pre-dehydrated gas is conducted through contained filled with a molecular sieve. Water molecules are held back by the molecular sieve. Certain molecular sieves can also remove hydrogen sulphide or hydrocarbons.
Particles of dirt are also separated out in this first step in the gas cleaning process.
Step 2: Removing the acid, corrosive gases hydrogen sulphide and carbon dioxide (acid gas removal, AGR)
The Claus process, a combination of a physical and chemical process, is suitable for separating out hydrogen sulphide. Hydrogen sulphide is firstly selectively scrubbed from the natural gas (e.g. using diisopropylamide during "amine scrubbing"). It can be released again by heating and catalytically burned to water and sulphur. The sulphur is often used commercially. Even after passing through the Claus process, the natural gas still contains traces of various odorous sulphur compounds. Many processes are suitable for fine desulphurisation. Generally, the components are hydrated to form hydrogen sulphide, which in turn is removed using amine scrubbing. The last remnants are catalytically or thermally converted to sulphur dioxide.
Carbon dioxide can be bound in various solvents. Alternatively, for natural gas rich in carbon dioxide, a cryogenic process is chosen whereby the carbon dioxide is condensed together with heavy hydrocarbons in what is known as a cold box.
Step 3: Removing nitrogen and helium
In a nitrogen separation plant, the natural gas and any helium it may contain are usually partially condensed and separated using distillation columns. This generally occurs directly as part of the LNG liquefaction process. However, it also works without a cryogenic process – using a two-stage membrane process, with which valuable, highly pure helium in particular can be recovered.
Step 4: Mercury
When dealing with raw natural gas, which contains large quantities of mercury, the mercury is also separated out, generally using filtering plants. Various adsorption materials are used in this process, such as a mixed metal oxide with a dispersed, active copper sulphide phase.
The raw natural gas (feed gas) can, of course, only be cleaned immediately prior to gas liquefaction as described above. Sometimes, however, initial preparation already occurs before transporting to the liquefaction plant.
Liquefying cleaned natural gas
LNG is actually produced by deep cooling in what is known as the LNG train, the gas liquefaction plant. However, the aforementioned natural gas still always contains additional hydrocarbons, particularly ethane, propane and butane. The actual LNG liquefaction plant is therefore upstream of the pre-cooling process. Using a refrigerant (propane) at -35 °C, hydrocarbons from pentane and higher (i.e. with five or more carbon atoms) are separated out in order to prevent them separating by freezing during the methane liquefaction process.
Carl Linde lays the foundations
The actual deep cooling then follows, a process for gas liquefaction in heat exchangers, which is consistent with the air liquefaction process developed by Carl von Linde (the Linde process). The process uses the Joule-Thomson effect: If a condensed gas expands, its temperature changes. When the Joule-Thomson coefficient is positive, it cools down.
During compression, however, the gas temperature increases. This is why the gas being compressed must be cooled. Methane gas, ethylene or nitrogen that has already been cooled is used to do so. Very low temperatures can be achieved by alternating expansion, compression and cooling under constant pressure. In the production of LNG, the almost pure methane gas is cooled over several steps to a temperature between -162 °C and -167 °C; the exact temperature at which the phase transition from gas to liquid occurs depends on the purity of the gas. This liquefaction of natural gas is particularly efficient using multi-stage cooling processes with mixed refrigerants.
LNG is stored as a cryogenic liquid in well-insulated tanks.
Effective explosion protection for safe LNG production
As natural gas is easily combustible, safety measures must be put in place in all phases of production so as to reliably prevent fires and explosions. Natural gas and LNG are classified as explosion group IIA and temperature class T1 according to ATEX and IECEx.
Technical equipment, such as electric motors, must not ignite the gas. They are used to operate cooling machinery and heat exchangers, particularly in driving pumps and compressors. Vast amounts of energy are required here – around 10 to 25 per cent of the calorific value of the liquefied natural gas. Pumps and fans, driven by electric motors, are also required in the upstream processes for cleaning and transporting the natural gas.
For example, centrifugal or turbine pumps are used, which are fitted with explosion-protected motor actuators and power distribution boards. Various types of protection are possible, such as flameproof enclosure (Ex d), pressurized enclosure (Ex p), increased safety (Ex e) or encapsulation (Ex m) versions. Several degrees of protection can also be combined. In this way, actuators and power distribution boards can be configured which allow very high outputs with many current dependencies in the hazardous area. Better systems comprise Ex-protected remote I/O systems that allow access to fault dependencies of the pumps and power distribution boards via a distributed control system, thus making maintenance and overhaul more efficient and increasing the availability of the systems.
In addition to automation and low-voltage technology, Ex-protected technology is mandatory for system lighting too. A technically mature, innovative complete package for explosion protection makes the natural gas liquefaction process safe and efficient.
Further Reading:
These articles might also interest you
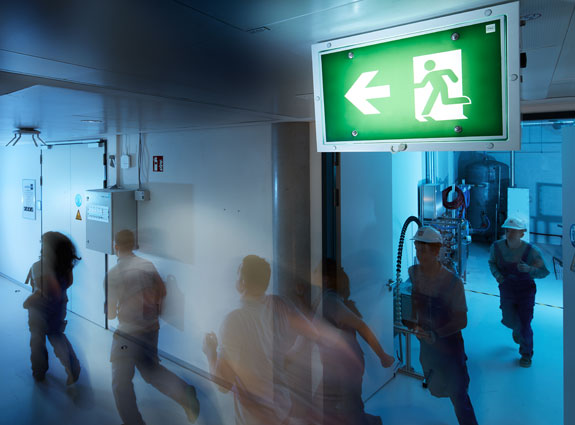
Central battery systems for standard-compliant emergency lighting
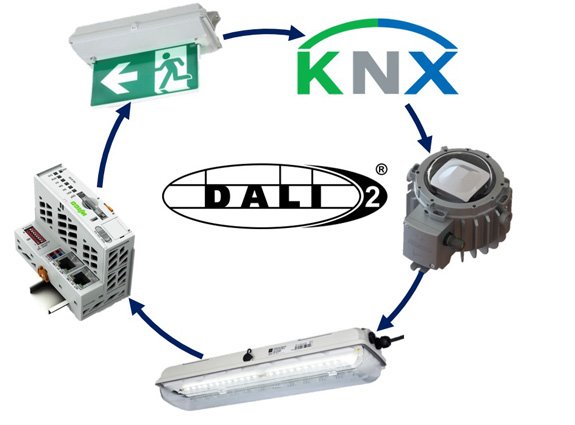
Write new comment