Surplus green electricity can be stored. This can be used by electrolysers to produce hydrogen. This power-to-gas method is expected to play a key role in successfully achieving our goal of climate-neutrality for the power supply sector. Development teams across the globe are currently working on improving the various possible electrolysis methods and scaling them up for use in megawatt- and gigawatt-scale electrolysers. These require maximum efficiency, safety and reliability. So what are the implications for explosion protection?
The history of water electrolysis goes way back. British chemists first succeeded in their attempt to split water into hydrogen and oxygen using electric current back in 1800. Around 40 years later, scientists managed to reverse this process, obtaining electricity from the reaction of the two gases – this is the basis of the fuel cell. But it was not until the start of the twentieth century that hydrogen first came under the spotlight as a useful chemical feedstock. It was at that time that the Haber-Bosch process was developed – a process that we have been using ever since to synthesise ammonia (and ultimately, fertiliser) from hydrogen and nitrogen. Coal gasification and steam reforming (using natural gas and water) have been the primary means of obtaining the large quantities of hydrogen that this process requires. These methods – unlike electrolysis – also release carbon dioxide as a byproduct, which is associated with negative impacts on the Earth's climate. Unfortunately, for a long time, it was the case that electrolyser technology had not matured sufficiently to represent a realistic economical and environmentally friendly alternative.
In the wake of the first oil crises, which occurred in the 1970s and 1980s, developers came up with the first concepts in which hydrogen served as an energy carrier. In projects such as Hysolar, hydrogen was produced using solar power. Although these processes were far from being economical, it is quite surprising that they did not pull out all the stops to optimise them, since people were already aware at that stage that fossil fuels are a finite resource and numerous experts had expressed their concern that the growing concentration of carbon dioxide in the atmosphere had reached extremely critical levels. Nevertheless, in 1994, the Hysolar partners (Saudi Arabia and Germany) presented the world's first hydrogen production technology to directly couple photovoltaics and water electrolysis.
Different water electrolysis methods
Now that we are seeing more and more wind turbines and photovoltaic installations being used to generate electrical energy, one thing is becoming increasingly clear: Hydrogen produced using surplus green electricity will have an important role to play in the transition to more sustainable energy practices. For the purpose of sector coupling, hydrogen can be used as a storage medium. Water electrolysis is based on an incredibly simple principle comprising two partial reactions, which differ depending on whether the solution used is acidic or alkaline. In acidic solutions, hydrogen and water form from hydronium ions (H3O+) at the cathode. At the anode, water is converted to oxygen and hydronium ions.
In contrast, the use of potassium hydroxide solutions or neutral salts as the electrolyte results in water being converted to hydrogen and hydroxide ions (OH-) at the cathode. At the anode, water reacts to form oxygen and hydronium ions.
Besides a cathode, anode, and a container for the electrolyte, the water electrolysers used for these processes also feature a membrane (diaphragm). This membrane separates the cathode and anode sections, and prevents the hydrogen and oxygen gases that are produced from coming into contact with one another, while selectively allowing the ions that are formed (H+ in acidic electrolysers, OH- in alkaline electrolysers) to pass through. This enables the electrical circuit to be closed.
There are now various types of water electrolysers with multiple electrolysis cells arranged in a stack. Aside from the kind of electrolyte used, they also differ in terms of the specific cell components (e.g. the membrane, gas diffusion layer (GDL), type of bipolar plates and catalysts) and in terms of the temperatures and pressures that occur during the electrolysis process. In essence, all electrolysers use direct current to split two water molecules into two hydrogen molecules and one oxygen molecule.
The following types of electrolyser are the most common:
AEL (alkaline electrolysers): These usually use potassium hydroxide solution as the electrolyte, coupled with nickel-based electrodes. Alternatively, titanium electrodes with a ruthenium oxide or iridium oxide coating can be used. AEL electrolysers are operated at temperatures of between 40 and 90 °C and pressures of between 1 and 30 bar. This type of electrolyser has been in use since the 1950s and boasts excellent long-term stability.
PEM (proton exchange membrane, acidic electrolysers): These use distilled or drinking water. A polymer membrane that is permeable to protons is used as the electrolyte. On the cathode side, it is coated with platinum/carbon, and on the anode side, it is coated with iridium oxide or ruthenium oxide. The bipolar plates are made from titanium. There are also reversible PEMs, which can be used as either fuel cells or electrolysers. When combined with a hydrogen storage system, this can be useful for energy storage purposes. PEM electrolysers are largely maintenance-free. Unlike AEL electrolysers, this type of electrolyser can go from standby mode to full-load operation in a matter of seconds. This is important particularly when it comes to storing wind energy.
SOE (solid oxide electrolysis, solid oxide electrolysers): The electrolyte is a ceramic material between the half-cells. Water is supplied in the form of steam. SOE electrolysers are operated at between 600 and 900 °C (high-temperature electrolysis) and are highly efficient (over 80% efficiency), partly due to the excess energy that is coupled in as heat.
AEM (anion exchange membrane): These feature an ion-conducting membrane, which is coated with catalyst on both sides. In contrast to PEM electrolysers, hydroxide ions (OH-) pass through this membrane. The membrane in the more recently developed AEM electrolyser is significantly more cost-effective than that of the PEM electrolyser. It is sandwiched between gas diffusion layers. The end plates on both sides of the assembly are steel bipolar plates. Their efficiency rating is 62.5%. The hydrogen that is produced is 99.9% pure.
The risks associated with hydrogen are manageable
From a safety perspective, all the processes are comparable. When it is produced, the hydrogen initially takes the form of gas dissolved in water. If any of the electrolyte manages to leak out, this may result in hydrogen being released. Hydrogen can combine with the oxygen that is also produced during the process and/or with the ambient air to create an extremely explosive mixture that spans a very wide explosive range. Mixtures with a content between 4 vol% and 77 vol% can explode. Only a tiny amount of ignition energy – 0.02 mJ – is required in order to trigger an explosion. This has earned hydrogen its place in the most hazardous ignition group, IIC. The extremely high flame propagation speed makes hydrogen explosions incredibly destructive.
Over 100 years' experience with hydrogen have given the chemical industry a good grasp of these associated risks and how to mitigate them. Explosion protection is currently regulated by standards series IEC 60079 and IEC 80079. The requirements pertaining to the safe construction and operation of electrolysis systems are set out in international standard ISO 22734.
Primary explosion protection through appropriate design and construction
The clear priority here is to prevent an explosive mixture from being able to occur in the first place. Taking measures to ensure that the entire system is leak-tight to prevent hydrogen from escaping is one of the pillars of primary explosion protection. Gas sensors in the surrounding area will sound the alarm even if only minuscule levels of hydrogen are detected. The importance of ensuring that these primary explosion protection measures are in place cannot be overstated. Measuring a mere 0.276 nm, hydrogen molecules are incredibly small and can diffuse through many metal materials. This can make metals brittle. As a result, the task of making equipment and pipe joints leak-tight is more complex and laborious than for most other processes. Strict requirements apply, notably for high-temperature processes such as SOE, which take place at temperatures exceeding the minimum ignition temperature of hydrogen (585 °C). Measures must also be taken to prevent the hydrogen that forms from being contaminated by oxygen.
Zone classification and secondary explosion protection
System manufacturers are required to conduct risk assessments – these assessments systematically identify potential hazards, along with the requisite countermeasures. The safety strategy must be based on the zone classification system set out in IEC 60079-10-1 – the operating company is responsible for this. It is necessary to take into account not just the electrolyser, but also the downstream gas conditioning system, any compressors, conduits and storage systems. Zones characterise hazardous areas based on the duration and frequency with which a hazardous explosive atmosphere (HEA) may occur:
Zone 0: A place in which a HEA is present continuously or for long periods or frequently.
Zone 1: A place in which a HEA is likely to occur in normal operation occasionally.
Zone 2: A place in which a HEA will not or is not likely to occur in normal operation but, if it does occur, will persist for a short period only.
This zone classification system forms the basis for secondary explosion protection, which focuses on the prevention of ignition sources. One example of a critical Zone 2 area might be the area directly below the ceiling in rooms in which electrolysers are being operated. Whether this area actually needs to be classified as Zone 2 depends on whether other, mitigating organisational and technical measures are in place. For instance, providing forced ventilation in this location may prevent hydrogen from accumulating there to critical, explosive levels. Since gaseous hydrogen dissipates very quickly, this is generally sufficient to minimise the risk of explosion. Consequently, this area does not need to be given a zone classification.
Different approaches for low- and high-pressure electrolysers
The above is normally the case for atmospheric electrolysis systems with operating pressures of no more than 50 mbar, provided hydrogen leakage is limited to only negligible amounts. This standard must be consistently maintained by regularly monitoring and checking the equipment, and replacing components such as seals as necessary. Additionally, gas detectors are a safeguard that issues an immediate alert in the event that the hydrogen concentration in the room reaches impermissible levels.
For high-pressure electrolysis systems with operating pressures of up to 100 bar, these gas detectors are crucial. If the circumstances demand it, they may need to shut down the system prematurely. Generally speaking, an area measuring 0.5 m around the cell block and extending up to the ceiling is classified as Zone 2. Although high-pressure systems are energetically favourable, they necessitate precise knowledge of the effects of pressure and temperature on the hydrogen-oxygen system's explosion limits. Experts must also carefully ascertain permeation and leakage rates. To prevent a hazardous situation arising in which the hydrogen is contaminated with oxygen (e.g. because the gases were improperly separated during the process), the electrolyser must be operated with due care.
ATEX-certified equipment in Zones 0 to 2
It goes without saying that only suitable equipment that has the appropriate certification may be used in areas classified as Zones 0 to 2. Portable devices should ideally be suitable for Zone 0. This guarantees maximum safety, even if employees would normally only remain in Zone 2 when walking around the plant. Operating panels for Zones 1 and 2 and explosion-protected panels with Ex d or Ex e enclosures may be installed near the electrolysers. Relay modules and transmitter supply units should also have the requisite ATEX certification for Zone 1 or 2. Control cabinets may also be equipped with a ventilation system to prevent hydrogen from accumulating to critical levels.
Summary and future developments: Complete explosion protection for gigawatt-scale systems
Hydrogen production is subject to stringent regulations. Special requirements pertaining to primary, secondary and tertiary explosion protection apply to the electrolysers and other apparatus in the hydrogen system, depending on what type they are, how they are constructed and how they operate. The stringent safety requirements also apply to pumps for delivering water and/or electrolyte, gas dryers and a whole host of other devices. Additionally, measures must be taken to ensure that the products used for supplying power to and controlling the systems, along with any fans, light fittings, sensors and many other installations, operate safely and reliably at all times, and that, should a hydrogen-air mixture form, they cannot ignite it. Electrolysis systems that are destined for mass production must be designed with particular care. Aside from the safety considerations, their reliability must also be optimised. This sets the stage for the future production of modular, gigawatt-scale systems that will play a significant part in supplying power safely and reliably using green, climate-neutral technologies.
Further Reading:
These articles might also interest you
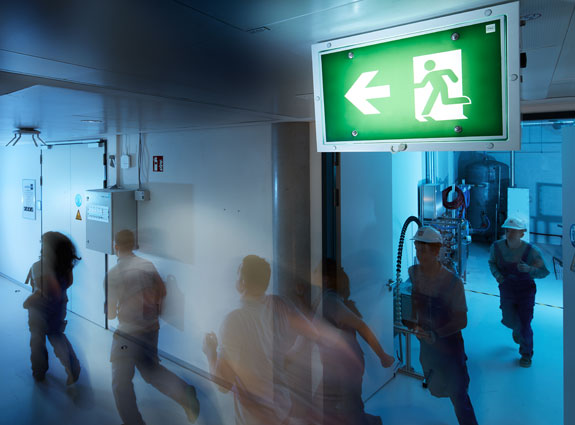
Central battery systems for standard-compliant emergency lighting
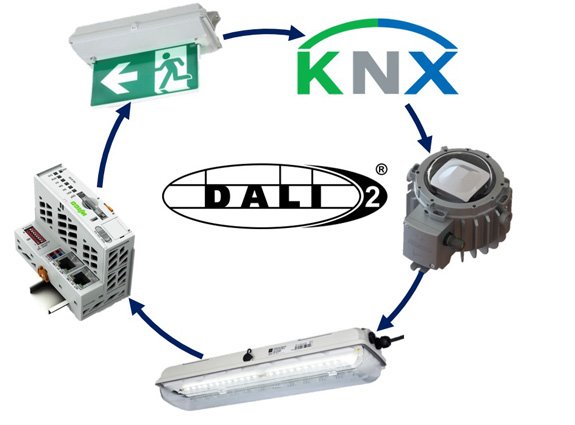
Write new comment